Escherichia coli as a Model Organism (chapter three)
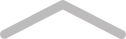
Escherichia coli as a Model Organism (chapter three)
The synthesis of group A colicins is a lethal event for the cell. After induction, the number of viable cells immediately starts to decrease while the amount of colicin is increasing. Cell death is due to the colicin lysis protein coexpressed with colicin. Its killing process against its host is unknown. It seems to be responsible for the shutoff of chromosomal protein synthesis reported during colicin induction.Colicin operons of group B colicins contain a lysis gene when carried by plasmids of type I, as colicins 5 and 10, but do not contain a lysis gene when present on type II plasmids, except that of colicin D. Thus, their synthesis is not lethal for the producing cells and is not followed by the release of colicin into the extracellular medium.
Colicins, once the model for studies of bacterial toxins, has significantly contributed to progress in a number of fields. The fascinating aspect of colicin is the specificity and the diversity of interactions that it undergoes with various proteins under the course of its action and during its production and release. A great number of these interactions with proteins of the different cell compartments has been demonstrated by genetic, biochemical, and structural investigations and analyzed in vivo and, sometimes, in vitro, but many of them have yet to be deciphered. The most intricate association undertaken by colicin is the association with its cognate immunity protein, which has been extensively studied in the case of nuclease colicins. The specific associations of colicins with the outer membrane protein receptors, the different proteins of the translocation machinery, and the inner membrane immunity proteins have also been well characterized, and their studies are progressing rapidly. Among the various colicin processes that warrant further investigations are mainly the dissociation of the complex nuclease colicin immunity protein, the physiological role of the Tol system, the entry of nuclease colicin into the cytoplasm, the transformation of pore-forming colicins from soluble proteins to membrane proteins, the structure of the voltage-gated channels that they form, and the mechanism of colicin export. In the next 80 years, many answers will be found, more colicins will be isolated, more colicin interactions will be known, and more than the current application of colicin and colicin cleavage protein in genetic engineering will be realized.
2. Inner membrane protein
Membrane proteins interact with, or are part of, biological membranes, and they separate the cytoplasm from the extracellular environment in all living cells. These membrane proteins are prevalent as they are encoded by 20 to 30% of all genes in most genomes. For Escherichia coli, the inner membrane is a phospholipid bilayer, and integral inner membrane proteins are mostly α-helical. The inner membrane proteins include transporters, channels, receptors, enzymes, and structural membrane-anchoring domains for myriad tasks, including energy transduction and cell adhesion. Most proteins are inserted into the membrane by the well-conserved Sec pathway, consisting of the membrane-spanning translocase SecYEG in bacteria. Many accessory proteins, including the signal recognition particle (SRP) and its cognate membrane receptor FtsY, aid in protein targeting and insertion. To be tagged to the membrane via the Sec pathway, a protein usually has an N-terminal signal sequence for recognition by the SRP. Translation of the N-terminal signal peptide that target proteins to the SRP pathway is required for directing mRNAs to the membrane. In E. coli, YidC protein can be specifically cross-linked to the transmembrane domain of newly synthesized peptides during their membrane insertion via translocons (complexes of proteins associated with the translocation of polypeptides across membranes). Several lines of evidence have revealed that in E. coli, as well as in mammalian cells, ribosomes translating inner membrane proteins interact cotranslationally with translocons in the membrane, and this interaction is required for the proper insertion of nascent polypeptides into the membrane. C-tail-anchored inner membrane proteins, a class of proteins characterized by their lack of N-terminal signal sequences, were first identified in eukaryotes, and they play critical roles in membrane traffic, apoptosis, and protein translocation in eukaryotes. Tail-anchored inner membrane proteins have been found in Streptomyces coelicolor, and they are capable of targeting proteins to the membrane in the absence of an N-terminal signal sequence; the C-terminal transmembrane domain is sufficient for membrane targeting. By considering the distance of the transmembrane domain from the C terminus (less than 30 residues) and also the lack of an N-terminal signal peptide, 12 proteins were identified as C-terminal-anchored proteins in E. coli. These 12 proteins include those known to be associated with the inner face of the cytoplasmic membrane, such as the flagella assembly protein FlK, the TraL protein involved in F pilus formation, and enzymes with hydrophobic substrates that are expected to be favored by membrane anchorage. Only three of the 12 C-tail-anchored proteins, namely, YgaM and paralogs ElaB and YqjD, have not been characterized. Recently, these three proteins were shown to be associated with stationary-phase ribosomes. Complexes in the inner membrane of E. coli are often involved in key processes, such as energy generation, cell division, signal transduction, and transport. Targeting small stress resistance proteins is an emerging area for treating bacterial infections; however, there are risks in targeting YgaM, ElaB, and YqjD as their function is not known.
ElaB is a small protein of 101 amino acids. It has one transmembrane domain at the C terminus, and this domain is conserved in several bacterial species, including opportunistic pathogens. By contrast, the N termini of these proteins show much less conservation in terms of length and amino acid composition. Two proteins in E. coli, YqjD and YgaM, have C-terminal transmembrane domains that are the same as in ElaB. Moreover, the transmembrane domain is very close to the end of the C terminus and is followed by two to four residues containing one to three arginines. Inner membrane proteins can mediate antibiotic resistance as well as persister cell formation either by damaging the cell membrane or by affecting membrane permeability. Persister cells are phenotypic variants of regular cells, play a major role in the high antibiotic resistance of bacterial biofilms, and are likely responsible for the recalcitrance of chronic infections to antibiotics.
Bacterial membranes play essential roles in the responses to various stresses. Two small hydrophobic polypeptides, TisB and GhoT, are toxin components of toxin-antitoxin systems. Neither of these is under the regulation of RpoS, but they are found to increase persister cell formation by reducing cell metabolism to create dormancy. GhoT is a small membrane protein with two transmembrane domains (residues 7 to 27 and 37 to 57). Additionally, TisB and GhoT damage cell envelopes and lead to cell death when overproduced, but overproduction of ElaB did not cause growth inhibition, indicating that different inner membrane proteins and transmembrane proteins may function differently in the presence of general stress. By using in vivo fluorescence imaging and next-generation sequencing, bacterial persister cells, in addition to dormancy, employ an “active defense” to pump antibiotics out and reduce intracellular drug concentrations through enhanced efflux activity. Since ElaB does not seem to affect the metabolic state of the cell, it is possible that the function of ElaB is involved with efflux pumps either by enhanced pumping of the antibiotic out of the cell or by limiting the uptake of the antibiotic. Although the C-terminal transmembrane domains of ElaB and YqjD are highly conserved, the functions of ElaB and YqjD seem different. YqjD inhibits cell growth by binding to the 30S subunit in the 70S and 100S ribosomes at the N-terminal region. However, the N-terminal regions of these two proteins are not highly conserved, and overproduction of ElaB does not lead to growth inhibition or cell lysis.
References
[1] Lourenço A, Carneiro S, Rocha M, et al. Challenges in integrating Escherichia coli molecular biology data[J]. Briefings in Bioinformatics, 2011, 12(2):91-103.
[2] Houten B V. Nucleotide excision repair in Escherichia coli. [J]. Microbiological Reviews, 1990, 54(1):18-51.
[3] Beloin C, Roux A, Ghigo J. Escherichia coli biofilms. [J]. Curr Top Microbiol Immunol, 2008, 322(6):249-289.
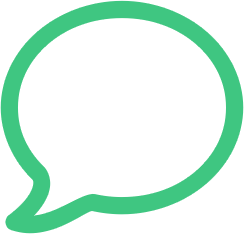