The new chapter in tumor immunotherapy—immunological checkpoint inhibitors (part two)
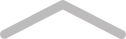
The new chapter in tumor immunotherapy—immunological checkpoint inhibitors (part two)
2. Biomarkers related to the efficacy of immunological checkpoint inhibitors
2.1. Immune related biomarkers
In view of the anti-PD-1/PD-L1 antibody prediction, the expression of PD-L1 in tumor tissues has become the primary research object. The clinical research results confirm that the expression of PD-L1 in tumor tissues is closely related to the efficacy of tumors on immunological checkpoint inhibitors. In addition, studies have found that the increase of CD8-positive T cells infiltrated in the tumor microenvironment and the secretion of γ-interferon often suggest that the tumor causes an active immune response, and the immune checkpoint inhibitor often has a better response rate for treating tumors; and the infiltration of regulatory T cells in the tumor microenvironment, overexpression of other immune checkpoint molecules such as TIM-3, LAG3 and IDO suggests that the tumor may have other immune escapes other than the PD-1/PD-L1 signaling pathway, under the circumstances, immune checkpoint inhibitor treatment response may be poor. It was found that the CC family chemokine ligand 9 (CXCL9) was inhibited by epigenetic modification and its biological function was inhibited, which eventually blocked the infiltration of effector T cells into the tumor bed to exert its immune function. For this reason, when a single immunological checkpoint inhibitor is insufficient to inhibit tumor growth, the combination of other immunological checkpoint inhibitors and epigenetic modification inhibitors may enhance the tumor killing effect.
Although the above immune molecules are indeed associated with the efficacy of immunological checkpoint inhibitors, they are also insufficient as biomarkers. Taking the study of PD-L1 molecules as an example, firstly, the expression of PD-L1 molecules in the tumor microenvironment shows dynamic diversity: it is caused by chromosome alteration, such as 9p24.1 gene translocation and amplification; or it is caused by activation of oncogene signaling pathways such as mitogen-activated protein kinase (MAPK), phosphatidylinositol 3-kinase/protein kinase B (PI3K/AKT); or it is caused by other immune cell molecules such as γ-interferon. Inducible expression. For this reason, PD-L1 expression detection at a single time point does not assess tumor response to immune checkpoint inhibitors. Secondly, the differences in PD-L1 detection methods, threshold setting of expression, and tumor heterogeneity have not yet become reliable biomarkers for the efficacy of immunological checkpoint inhibitors. Moreover, its sensitivity and specificity for predicting the efficacy of immunological checkpoint inhibitors are somewhat insufficient. Subgroup analysis showed that the response rate of tumor immunological checkpoint inhibitors with positive PD-L1 expression was 36% to 100%, while negative PD-L1 expression was 0-17%. Therefore, in order to reduce the inaccuracy of single immune molecule detection, it may be more valuable to jointly detect multiple immune molecules.
2.2. Genomics-related biomarkers
With the development of gene sequencing and bioinformatics, the use of genomics technology to deeply analyze tumor tissue samples sensitive or resistant to immunological checkpoint inhibitors has become an important method for discovering biomarkers. Clinical studies have shown that microsatellite instability or mismatch repair defects may be responsible for the rate of immunological checkpoint inhibitors, and immunological checkpoint inhibitors have been approved by the US FDA for the treatment of such genomic abnormalities. In previous studies, the researchers reported that a patient with endometrial cancer was sensitive to immunological checkpoint inhibitors, by analyzing the genomic data of tumor primary and metastatic lesions, researchers found that the DNA polymerase ε (POLE) gene mutation may be related to this phenotype, probably because the mutation of the POLE gene affects the stability of the microsatellite. In addition, non-small cell lung cancer patients with TP53, KRAS gene mutations responded better to immunological checkpoint inhibitors, probably because TP53 and KRAS mutations changed a series of genes expression, including cell cycle regulation, DNA replication, and damage repair, it also can synergistically activate the expression of PD-L1. In addition, studies have found that patients with homozygous human leukocyte antigen class I (HLA-I) loci A, B, and C have better clinical benefit than patients who are homozygous for a single site for immunology inhibitor therapy.
The discovery of a single gene or a class of genes prompted researchers to perform a more systematic analysis of tumor samples. The researchers performed whole-exome sequencing of tumor tissue samples in patients with non-small cell lung cancer treated with immunological checkpoint inhibitors, and found that tumor tissue samples from patients with better response to immunological checkpoint inhibitors often have higher non-synonymous mutation. The researchers performed genome sequencing on pre-treatment biopsy specimens of melanoma immunological checkpoint inhibitors and paired paracancerous tissue samples, and found that the number of tumor somatic mutations was positively correlated with the efficacy of immunological checkpoint inhibitors. In addition, renal cancer patients who respond better to immune checkpoint inhibitors often have more mutations such as gene insertions and deletions than tumor tissues that are insensitive to immunological checkpoint inhibitors. Based on those, the tumor mutation load has been more widely studied than the relationship between single gene and immune checkpoint inhibitors. Tumor mutation load analysis of different tumor types based on large databases found that cancers with higher tumor mutation load, such as melanoma, non-small cell lung cancer, head and neck squamous cell carcinoma and bladder cancer, have higher response rates to immune checkpoint inhibitors. However, those cancers with low tumor mutation load, such as pancreatic cancer and prostate cancer, have a poor response rate to immune checkpoint inhibitors. Researchers speculate that this finding is closely related to tumor neonatal antigens, which are tumor cell gene mutations or rearrangements, insertions and deletions, and other gene expressions that specifically express on the surface of tumor cells, and are also target for specific recognition and attack of T cells in the body's immune response. The higher the tumor mutation load, the more likely it is to express a specific antigen that can be recognized by immune cells. At this time, the use of immunological checkpoint inhibitors tends to have better anti-tumor effects.
However, there are several questions about the tumor mutation load as a biomarker for predicting the efficacy of immunological checkpoint inhibitors. First, there is no exact tumor mutation load threshold to determine which population can be obtained benefit from immunological checkpoint inhibitor treatment. Secondly, the tumor neonatal antigen that causes the immune response attack may be only a small part of the gene mutation. Detection directly conducted on the surface of tumor antigen load at the protein level may be more accurate to predict the tumor immunoassay effect. Thirdly, tumors with low tumor mutation load may enhance the immunogenicity of their tumor neonatal antigens by epigenetic modification, and the therapeutic response of immunological checkpoint inhibitors is better, and those with high tumor mutation load in the tumor immune microenvironment may also have other immunosuppressive molecules, such as interleukin 10 (IL-10), metabolic related enzymes IDO and others. Therefore, the use of tumor mutation load alone to predict the efficacy of immunological checkpoint inhibitors may have certain deficiencies.
To be continued in Part Three…
Reference
1.Robert C, Thomas L, Bondarenko I, et al. Ipilimumab plus dacarbazine for previously untreated metastatic melanoma. N Engl J Med. 2011;364(26):2517-2526.
2.Dunn GP, Bruce at. Ikeda H, et al. Cancer immunoediting: from immunosurveillance to tumor escape. Nat Immunol. 2002;3(11):991-998.
3.Armand P, Shipp M A, Ribrag V, et al. Programmed death-1 blockade with pembrolizumab in patients with classical Hodgkin lymphoma after brentuximab vedotin failure. J Clin Oncol. 2016;34(31):3733-3739.
4.Chen D S, Mellman I. Oncology meets immunology: the cancer-immunity cycle. Immunity. 2013;39(1):1-10.
5.Zou W. Immunosuppressive networks in the tumour environment and their therapeutic relevance. Nat Rev Cancer. 2005;5(4):263-274.
6.Harrington K J, Ferris R L, Blumenschein G Jr, et al. Nivolumab versus standard, single-agent therapy of investigator's choice in recurrent or metastatic squamous cell carcinoma of the head and neck (CheckMate 141): healthrelated quality-of-life results from a randomised. phase 3 trial. Lancet Oncol. 2017;18(8):1104-1115.
7.Wang Q, Wu X. Primary and acquired resistance to PD-1/PDL1 blockade in cancer treatment. Int Immunopharmacol. 2017;46: 210-219.
8.Hanahan D, Weinberg R A. Hallmarks of cancer: the next generation. Cell. 2011;144(5):646-674.
9.Motzer R J, Escudier B, Mcdermott D F, et al. Nivolumab versus everolimus in advanced renal-cell carcinoma. N Engl J Med. 2015;373(19):1803-1813.
10.Topalian S L, Hodi F S, Brahmer J R, et al. Safety. activity. and immune correlates of anti-PD-1 antibody in cancer. N Engl J Med. 2012;366(26):2443-2454.
11.Thommen D S, Schreiner J, Müller P, et al. Progression of lung cancer is associated with increased dysfunction of T cells defined by co-expression of multiple inhibitory receptors. Cancer Immunol Res. 2015;3(12):1344-1355.
12.Tumeh P C, Harview C L, Yearley J H, et al. PD-1 blockade induces responses by inhibiting adaptive immune resistance. Nature. 2014;515(7528):568-571.
13.Topalian S L, Drake C G, Pardoll D M. Immune checkpoint blockade: a common denominator approach to cancer therapy. Cancer Cell. 2015;27(4):450-461.
14.Le D T, Uram J N, Wang H, et al. PD-1 blockade in tumors with mismatch-repair deficiency. N Engl J Med. 2015;372(26):2509-2520.
15.Pardoll D M. The blockade of immune checkpoints in cancer immunotherapy. Nat Rev Cancer. 2012;12(4):252-264.
16.Reck M, Rodriguez-abreu D, Robinson A G, et al. Pembrolizumab versus chemotherapy for PD-L1-positive non-small-cell lung cancer. N Engl J Med. 2016;375(19):1823-1833.
17.Brahmer J R, Tykodi S S, Chow L Q, et al. Safety and activity of anti-PD-L1 antibody in patients with advanced cancer. N Engl J Med. 2012;366(26):2455-2465.
18.Nghiem P T, Bhatia S, Lipson E J, et al. PD-1 blockade with pembrolizumab in advanced Merkel-cell carcinoma. N Engl J Med. 2016;374(26):2542-2552.
19.Patel S P, Kurzrock R. PD-L1 expression as a predictive biomarker in cancer immunotherapy. Mol Cancer Ther. 2015;14(4):847-856.
20.Restifo N P, Smyth M J, Snyder A. Acquired resistance to immunotherapy and future challenges. Nat Rev Cancer. 2016;16(2):121-126.
21.Postow M A, Callahan M K, Wolchok J D. Immune checkpoint blockade in cancer therapy. J Clin Oncol. 2015;33(17):1974-1982.
22.Meng X, Huang Z, Teng F, et al. Predictive biomarkers in PD-1/PD-L1 checkpoint blockade immunotherapy. Cancer Treat Rev. 2015;41(10):868-8
23.Dong Z Y, Zhong W Z, Zhang X C, et al. Potential predictive value of TP53 and KRAS mutation status for response to PD-1 blockade immunotherapy in lung adenocarcinoma. Clin Cancer Res. 2017;23(12):3012-3024.
24.Martens A, Wistuba-Hamprecht K, Geukes Foppen M, et al. Baseline peripheral blood biomarkers associated with clinical outcome of advanced melanoma patients treated with ipilimumab. Clin Cancer Res. 2016;22(12):2908-2918.
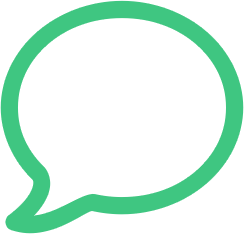